Summary: One of the fascinating aspects of plants is how the remarkable diversity of shapes and sizes of plant organs is obtained exclusively through the growth and division of pressurized cells that are surrounded and glued together by strong cell walls. In this respect, a central question, which applies to all walled organisms, is how cells can expand while maintaining the mechanical integrity of their cell walls. Answering this simple question is extremely challenging, not only due to the daunting complexity of the cell wall polymer networks but also because it requires integrating processes occurring at multiple spatial (molecule, wall, cell, organ, plant) and temporal (from milliseconds to days) scales. As a result, despite often detailed knowledge of growth-controlling signaling networks, we actually don't know on which critical cell wall-associated physicochemical processes they impinge, simply because we still mostly remain in the dark on what they are. This is a major obstacle to reliably model and predict plant growth responses to changing environments. The walls of growing plant cells mostly consist of carbohydrate polymers, notably cellulose and the matrix polymers hemicelluloses and pectins, with small amounts of glycoproteins. Changes in the polymer composition and architecture are known to occur during cell expansion, but it is often impossible to distinguish cause from consequence because of the presence of feedback mechanisms that monitor cell wall properties and elicit compensatory modifications. Resolving these issues requires new methods to (i) observe and quantify changes in polymer chemistry and architecture in intact growing cell walls and (ii) simultaneously perturb these changes and observe the cellular consequences at the appropriate time scales.
Plant cell wall expansion models So far, the biochemical events leading to cell wall growth were described by “biomechanical hotspot”, and “Ca-pectate exchange” models, respectively. The “biomechanical hotspot” model posits that cellulose microfibrils, with a small number cellulose-XG-cellulose cross-links form a load-bearing network and the removal of the crosslinks through the action of the wall loosening agents “expansins” at acidic pH promotes wall relaxation (Coen and Cosgrove, 2023). In the “Ca-pectate exchange” model, proposed for the freshwater algae Chara corallina and the tip-growing pollen tubes, chelation of Ca2+ from load bearing Ca2+-cross-linked pectate by newly produced pectate, replaces the load bearing cross links with new cross links, leading to cell wall relaxation (Boyer, 2016).
An alternative cell wall expansion mechanism Our team has recently formulated a new model of ‘intrinsic’ cell wall expansion in which the pectin homogalacturonan (HG) plays a critical role (Haas 2021). HG is a linear polymer of galacturonic acid (GalA), which is secreted in a methylesterified, uncharged form and, in the cell wall, can dynamically acquire negative charges by demethylation catalyzed by pectin methylesterases (PME). PMEs can demethylate HG randomly or in blocks. Genetic studies have shown that HG demethylation is essential for cell expansion, but the underlying mechanism was not clear. In this so-called “expanding beam” model, cell wall expansion is an emergent property of the insertion and modification of HG, resulting in a conversion of chemical into mechanical energy, permitting the growth. The model is based on observations with 3D direct Stochastic Optical Reconstruction Microscopy (dSTORM) nanoscopy that we developed in plants. This revealed, in certain cell walls, an unexpected architecture, in which pectin homogalacturonan (HG) is not part of an amorphous matrix, but forms organized nanofilaments oriented perpendicular to the growth axis. We proposed that the blockwise demethylesterification caused a volume increase corresponding to a phase transition in the HG filament fine-structure, driving the expansion of the cell wall. We also observed that randomly methylated HG did not form nanofilaments. Thus, the HG charge distribution is somehow correlated with the nature of the higher-order assemblies in accordance with in vitro studies. These observations illustrate the importance of knowing the nano- to meso-scale architecture of cell wall polymers and the need for tools to observe phase transitions in vivo for the formulation of plausible hypotheses on the growth mechanism.
A putative new growth sensing mechanism. Many tip-growing cells (e.g. root hairs) display fast oscillatory growth shadowed by temporal bursts in pH, cytosolic Ca2+, and ROS. The elucidation of these second-range pulsations, which point to timescales for the underlying molecular events, may contribute to understanding the growth enigma in general. A candidate cell wall sensing module involves plasma membrane CrRLKL1 (Catharanthus roseus Receptor Like Kinase Like 1) receptors for secreted Rapid Alkalinization Factor (RALF) peptides. RALF promotes the formation of a signaling complex consisting of the GPI anchored protein LLG1 and the receptor kinase FERONIA (Xiao et al., 2019), which appears to play a role in the regulation of the cell wall assembly process, e.g. indirectly leads to the alkalisation of the apoplast by the inhibition of the proton pump.
Cell wall patterning through peptide-protein-pectin interaction. The Most RALFs are polycationic, thus they are expected to interact with ‘undiffusable’ negative charges resulting from pectin demethylation. A multidisciplinary collaboration with Julia Santiago (UNIL), Kris Vissenberg and Bernard Cathala/Extelle Bonnin (BIA, Nantes), in part financed by ANR project HOMEWALL, ERC STORMtheWALL and Euro bioimaging grants, has led to the surprising finding that RALF peptides have both a signaling and a structural role in the cell wall. They form a complex with a cell wall protein Leucin Rich Repeat eXtensin (LRX), where the cationic peptide binds and condenses homogalacturonan into filaments that constitute a load bearing but extendable network (Moussu 2023). The binding of the free peptide to its plasma membrane receptor complex, appears to control the cell wall assembly process, through yet not a clear mechanism that we continue to investigate. These findings provide a biological rationale for this intriguing autocrine signaling system and shows many parallels with glycosaminoglycan-protein interactions in the animal extracellular matrix. This interaction causes the condensation of pectins, creating a reticulated pattern that confers resistance and extensibility to the wall (Moussu 2023). The collapse of the pectin matrix is accompanied by water loss, which shows that cell wall hydration is an important variable. This raises the interesting possibility that RALFs contribute to the growth (oscillations) by acting as a sensor for the cell wall charge density. In this hypothetical scenario, RALF binding to the receptor promotes wall alkalinization that activates PMEs (most PMEs have high pH optima), producing polyanionic HG. This, in turn, can sequester RALF from the receptor, causing wall acidification and PME repression until the next delivery of methylated HG and RALFs, closing the cycle.
Unravelling the key role of pH. In the acid growth theory, the hormone auxin activates the plasma membrane H+ATPase, causing wall acidification and cell wall relaxation by activating pH-sensitive wall loosening agents, such as expansins. The situation is more complex, however, since depending on the context, auxin can also promote cell wall alkalinization and HG demethylation and the latter was shown to be necessary and sufficient to promote cell wall expansion and organogenesis in a number of contexts. The work on root hairs shows that, at least in these cells, cell expansion is an oscillatory process in which the activities of pH-sensitive wall-modifying agents are expected to mirror the pH oscillations.
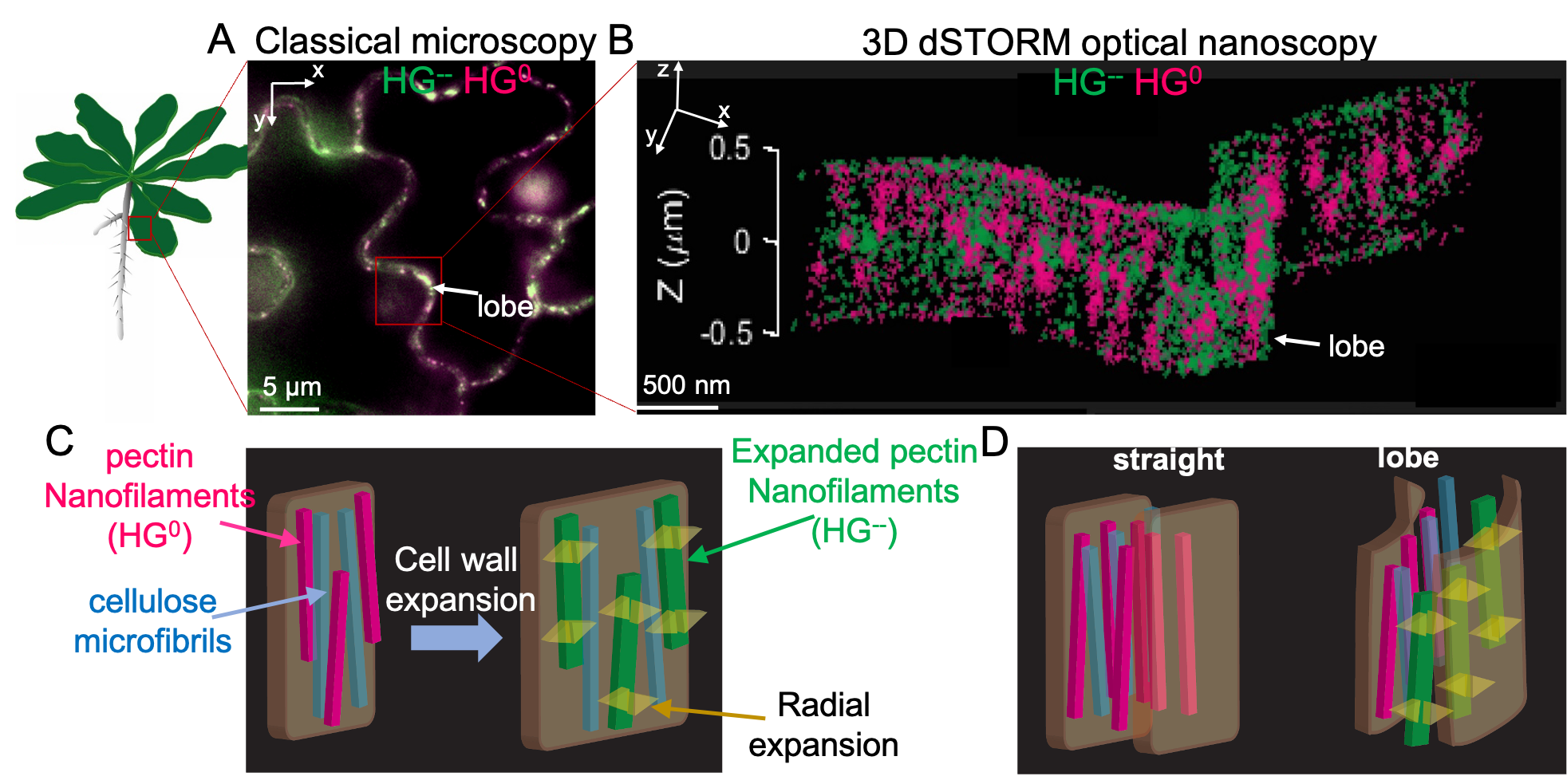
Figure 1. Morphogenesis through expansion of oriented nanofilaments. A) Classical fluorescence image of lobed epidermal pavement cells on the Arabidopsis Thaliana cotyledon. B) A super-resolved image of the lobed region highlighted in (A) with a red rectangle showing axially arranged HG nanofilaments. In (A) and (B) HG0 - methylated (neutral) pectin homogalacturonan, HG-- - unmethylated (polyanionic) pectin homogalacturonan C) A cartoon representation of the 'intrinsic cell wall expansion' through a pectin demethylation-driven nanofilament swelling. Pectin HG demethylation causes a radial swelling of the nanofilament and consequently cell wall expansion. D) Cartoon representation of the lobe formation through asymmetric HG demethylation along and across the cell wall.
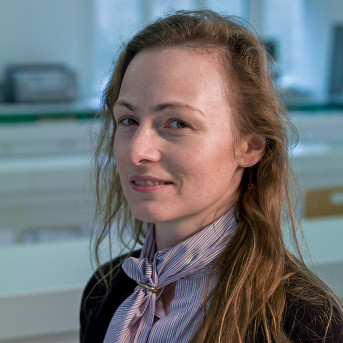